Applicable Regulations (FAA Part 107)
The Federal Aviation Administration (FAA) regulates the operation of small unmanned aircraft systems (sUAS) through Title 14 of the Code of Federal Regulations (CFR) Part 107, which is a crucial component for any remote pilot aspiring to fly drones commercially. This regulation outlines the specific rules that govern the certification process, operational limitations, and the privileges afforded to certified pilots. All sUAS flights that are conducted commercially or for non-recreational purposes must comply with Part 107 regulations, making this the foundation of legal drone operations in the United States.
Key Sections of 14 CFR Part 107:
- Certification: Section 107.73(a) requires remote pilots to pass the airman knowledge test to obtain certification.
- Operating Limitations: Includes altitude limits (400 feet AGL), speed (100 mph), and line-of-sight requirements.
- Airspace Restrictions: Drone operations are restricted in controlled airspaces without prior ATC authorization.
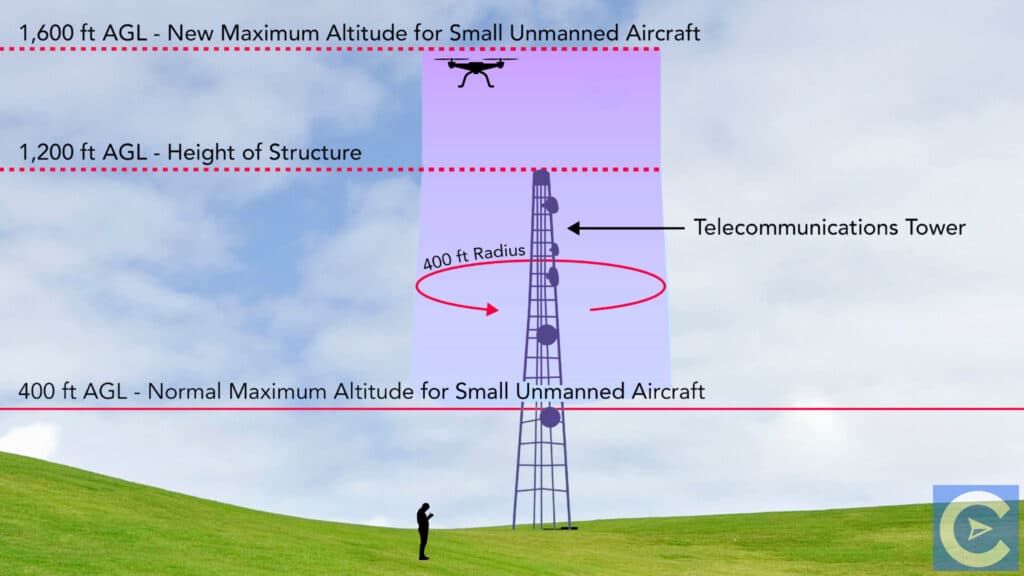
The regulations covered in Part 107 specifically provide the framework for the following key areas:
- Eligibility and Certification Requirements: Pilots must be at least 16 years old and pass an FAA-approved knowledge exam to become certified.
- Operational Limitations: These include flying under 400 feet above ground level (AGL), keeping the aircraft within visual line-of-sight (VLOS), avoiding flights over people unless authorized, and maintaining a safe distance from other aircraft and structures.
- Prohibitions on Hazardous Operations: Remote pilots must avoid careless or reckless operation of their aircraft, which could endanger people or property.
- Waivers: Certain sections of the regulations, such as night operations, may be waived if the remote pilot applies for a waiver and demonstrates that the operation can be conducted safely under alternative conditions.
Advisory Circular (AC) 107-2
The Advisory Circular (AC) 107-2 provides detailed guidance on the implementation of the regulations found in Part 107. It serves as a supplement to the regulations, explaining how to comply with the rules effectively. Remote pilots must be familiar with this AC as it is designed to clarify procedures, operational requirements, and safety standards.
Important Provisions in AC 107-2:
- Pre-Flight Assessment: Remote pilots are required to perform a pre-flight inspection, including checking the airspace, weather conditions, and ensuring the aircraft is in proper working condition.
- Maintenance Requirements: Routine maintenance checks should be performed to keep the drone airworthy.
- Communication with Air Traffic Control (ATC): AC 107-2 provides detailed guidance on how to communicate with ATC when requesting flight authorization in controlled airspace.
An example of AC 107-2 in action is a remote pilot conducting a visual line-of-sight flight near an airport. The pilot uses the circular to determine how to request clearance from ATC, ensuring that the operation complies with local airspace restrictions.
Airspace Classification, Operating Requirements, and Flight Restrictions
Airspace is categorized into two types: regulatory and nonregulatory. Within these categories, there are four types: controlled, uncontrolled, special use, and other airspace areas. The purpose of these distinctions is to ensure safety based on the complexity or density of aircraft operations, the level of safety needed, and national or public interest. Remote pilots must understand these categories to comply with regulations and ensure safe flights.
Controlled Airspace
Controlled airspace refers to areas where Air Traffic Control (ATC) services are provided. Remote pilots need to understand the following classes of controlled airspace that concern their operations:
- Class B Airspace: Generally extends from the surface to 10,000 feet mean sea level (MSL), surrounding the busiest airports. It resembles an upside-down wedding cake and is designed to contain all published instrument procedures. Remote pilots must receive ATC authorization to operate in Class B airspace.
- Class C Airspace: This airspace typically extends from the surface to 4,000 feet above airport elevation, surrounding airports with operational control towers and radar approach services. Remote pilots must receive authorization before operating in this airspace.
- Class D Airspace: Extends from the surface to 2,500 feet above airport elevation. This airspace surrounds airports with operational control towers. Remote pilots must receive ATC authorization before operating here.
- Class E Airspace: This is controlled airspace that is not classified as Class A, B, C, or D. It generally starts at 1,200 feet AGL, though it can start at lower altitudes in certain areas. Remote pilots typically do not need authorization to operate in Class E airspace unless specified.
Uncontrolled Airspace
- Class G Airspace: This is the airspace where ATC services are not provided, and it extends from the surface to the base of the overlying controlled airspace. Remote pilots can operate in Class G airspace without needing ATC authorization. This is the most common airspace for small unmanned aircraft operations due to its fewer restrictions.
Special Use Airspace
Special use airspaces are areas where certain activities must be confined, or where limitations on aircraft operations are imposed to separate potentially hazardous activities from civilian aircraft. These include:
- Prohibited Areas: Airspace where flight is completely prohibited for reasons related to national security or safety, such as around the White House or Camp David. These areas are marked as “P” followed by a number on aeronautical charts.
- Restricted Areas: Areas where operations may be hazardous to aircraft not participating in specific activities. An example might include areas where military firing or missile testing occurs. Remote pilots need permission to operate in these areas, which may be granted if the restricted area is not active.
- Warning Areas: Found over international waters, warning areas indicate areas where activities that might pose risks to nonparticipating aircraft occur. These areas are marked with a “W” and extend from 3 nautical miles outward from the coast of the United States.
- Military Operations Areas (MOAs): These separate military training activities from IFR traffic. MOAs are depicted on sectional charts, and remote pilots are advised to avoid operations in active MOAs unless proper clearance is obtained.
- Alert Areas: These areas alert pilots to unusual or high levels of aviation activity, such as pilot training. Remote pilots are responsible for maintaining collision avoidance.
Here is an example of prohibted area charted as a “P” followed by a number (P-40) and are circled by blue hash marks.
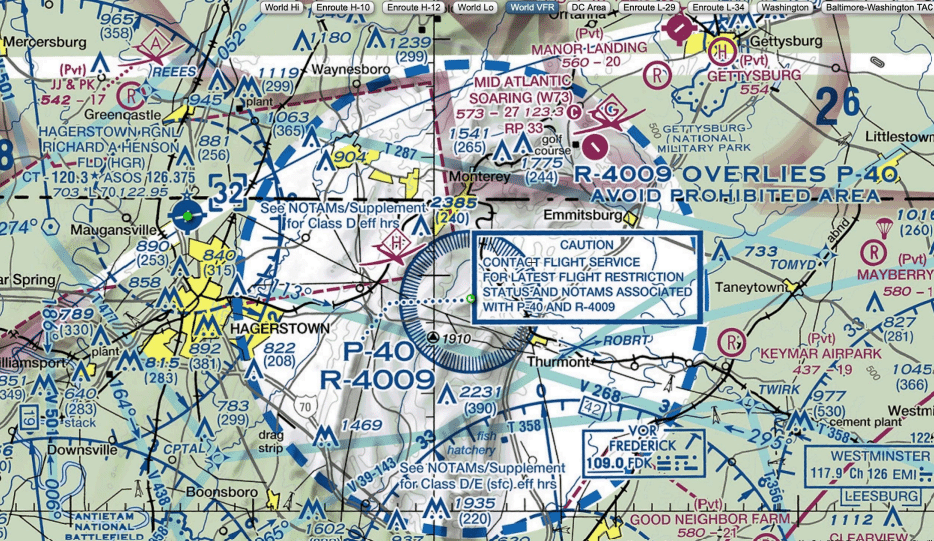
Other Airspace Areas
Other areas of importance include:
- Local Airport Advisory (LAA): Advisory services provided at airports without operating control towers, helping ensure safety for all aircraft in the vicinity.
- Temporary Flight Restrictions (TFRs): Designated by a Notice to Airmen (NOTAM), these restrictions protect people and property on the ground or in the air, often for reasons such as disaster relief, presidential movements, or public safety events. Remote pilots must check for TFRs as part of their pre-flight planning.
- Military Training Routes (MTRs): Established for military aircraft training, MTRs often involve high-speed, low-altitude flight. Remote pilots must avoid these areas unless they are inactive, as they pose significant risks for collision.
- National Security Areas (NSAs): Established to enhance security, particularly around sensitive infrastructure. Pilots are requested to avoid NSAs, though flight is not always strictly prohibited unless otherwise noted in a NOTAM.
Air Traffic Control (ATC) and the National Airspace System (NAS)
The primary function of ATC is to prevent collisions and organize traffic flow. Remote pilots interacting with ATC, especially in controlled airspace, must understand ATC communications and follow the procedures set forth to integrate unmanned aircraft into the NAS.
Aviation Weather Sources
Aviation weather services are provided through a network of government agencies and independent organizations, including the National Weather Service (NWS), Federal Aviation Administration (FAA), and Department of Defense (DOD). These services deliver weather forecasts and real-time data to pilots, enabling safe decision-making before and during flight operations. For remote pilots operating under Part 107, understanding these weather sources is crucial to ensure safety during small unmanned aircraft system (sUAS) flights.
Meteorologists rely on scientific research and computer modeling to predict weather patterns with increasing accuracy. These forecasts and observations, although not 100% accurate, provide vital information to pilots, allowing them to adjust flight plans based on current and predicted weather conditions.
Surface Aviation Weather Observations
Surface aviation weather observations offer a snapshot of current weather conditions at ground stations across the country. These stations are operated by both government entities and private contractors, providing continuous, up-to-date information. Remote pilots rely on this data to understand the local weather conditions at a specific airport or flight area.
Key weather sources include:
- Automated Weather Observing Systems (AWOS)
- Automated Surface Observing Systems (ASOS)
These automated systems compile local data, including wind speed, visibility, sky conditions, temperature, and altimeter settings. Surface observations cover small areas but provide highly relevant and localized information, which is particularly useful for drone operations in proximity to airports or controlled airspace.
Aviation Weather Reports
Weather reports provide current, accurate depictions of weather conditions. Some of the most important weather reports that remote pilots need to be familiar with are:
METARs: METARs are routine aviation weather reports that provide a standardized view of current surface weather conditions at an airport. They are updated regularly, and in cases of significant weather changes, a special METAR (SPECI) may be issued.
Components of a METAR:
- Type of Report: Routine METAR or SPECI (special report for changing conditions).
- Station Identifier: A four-letter code indicating the location, such as “K” for U.S. airports (e.g., KGGG for Gregg County Airport in Texas).
- Date and Time: The report’s timestamp in Zulu time (UTC).
- Wind: Speed and direction of wind in knots.
- Visibility: Reported in statute miles.
- Sky Condition: Cloud coverage, ceiling height, and cloud types.
- Temperature/Dew Point: Given in degrees Celsius.
- Altimeter Setting: Reported in inches of mercury, indicating atmospheric pressure.
PIREPs: Pilot Reports (PIREPs) are in-flight weather observations provided by pilots. They offer real-time updates about actual conditions encountered, which may not be fully reflected in forecasts.
Aviation Forecasts
In addition to real-time weather reports, aviation forecasts are essential for flight planning. These forecasts are based on observed weather and help predict future conditions for specific locations. The key forecast products that remote pilots should be aware of include:
- Terminal Aerodrome Forecasts (TAF): A TAF provides a forecast for a five-statute mile radius around an airport and is updated four times a day. These forecasts are especially useful for pre-flight planning, as they provide information about expected weather conditions, including wind, visibility, and sky conditions over a 24 to 30-hour period.
- Significant Meteorological Information (SIGMET): SIGMETs warn pilots about hazardous weather conditions such as severe turbulence, icing, or volcanic ash.
- Convective SIGMET (WST): Issued for severe thunderstorms, tornados, or hail. Remote pilots must avoid operations during convective SIGMETs due to the significant hazards they present.
Effects of Weather on Small Unmanned Aircraft Performance
Weather conditions have a direct and significant impact on the performance of small unmanned aircraft. The atmosphere’s characteristics can affect factors such as aircraft control, stability, and efficiency during flight. Understanding how weather elements such as pressure, temperature, and wind influence drone performance is essential for safe and effective operations.
Key environmental variables include density altitude, pressure, temperature, and humidity. Remote pilots must consider these factors when planning flights, as they directly affect the aircraft’s ability to operate efficiently and safely.
Density Altitude and Its Impact on Performance
Density altitude is a key concept when assessing aircraft performance in varying atmospheric conditions. Density altitude refers to the altitude at which an aircraft “feels” like it is flying, based on the air density present.
- High Density Altitude: When air density decreases due to factors like high altitude, high temperature, or high humidity, it leads to high density altitude. In these conditions, aircraft performance degrades because the air is less dense, which results in reduced lift and engine performance.
- Low Density Altitude: Conversely, low density altitude occurs in cooler, denser air at lower altitudes, where aircraft performance is generally better. Lower temperatures and higher pressures contribute to increased lift and engine efficiency.
Effects of Pressure on Air Density
Air pressure plays a critical role in determining air density, which in turn affects aircraft performance. Air density and pressure are directly proportional, meaning that as pressure increases, so does air density. Conversely, at lower pressures (such as at higher altitudes), air becomes less dense, which can negatively impact the performance of small unmanned aircraft.
Higher Altitudes: As air pressure decreases with altitude, drones will require more power to maintain the same performance as they would at lower altitudes. This also impacts the effectiveness of the aircraft’s propulsion system.
Effects of Temperature on Air Density
The temperature of the air also affects its density, with warm air being less dense than cold air. As a result, an increase in temperature reduces air density, leading to degraded aircraft performance.
- Hot Weather: In high-temperature environments, drones experience reduced lift and require more power to maintain altitude and stability. In these conditions, a drone’s battery life may be shortened due to the additional energy required for flight.
- Cold Weather: Conversely, cooler temperatures increase air density, allowing the drone to perform more efficiently, with improved lift and power consumption.
Effects of Humidity on Density Altitude
Humidity, or the amount of water vapor in the air, also influences air density. Moist air is lighter than dry air because water vapor is less dense than air molecules like nitrogen and oxygen. As the humidity level increases, the density of the air decreases, which can affect the drone’s performance.
- High Humidity: In high-humidity conditions, the drone experiences a higher density altitude, reducing overall performance. The aircraft will require more power to generate lift, and the effectiveness of its propulsion system may decrease.
- Low Humidity: In dry conditions, air is denser, contributing to better performance and more efficient flight operations.
Wind and Turbulence
Wind is a crucial weather element that impacts drone stability and control. Turbulence, which is caused by disruptions in the smooth flow of air, can significantly affect small unmanned aircraft.
- Wind Gusts: Sudden changes in wind speed and direction, known as gusts, can destabilize the drone. Remote pilots should be cautious when flying in gusty conditions, especially near ground obstructions like buildings or mountains, where wind flows can be erratic.
- Turbulence in Mountainous Regions: In areas with significant topography, wind currents follow the terrain, creating turbulence. When flying in mountainous regions, drones are subject to turbulent airflow on the leeward side of the mountains, which can push the aircraft down unexpectedly.
Low-Level Wind Shear
Wind shear refers to a sudden and drastic change in wind speed or direction over a short distance. Low-level wind shear is particularly hazardous, as it occurs close to the ground and can lead to sudden, unpredictable changes in the aircraft’s flight path.
For instance, a drone flying into a wind shear event could experience an abrupt loss of lift if a headwind changes to a tailwind. Conversely, a tailwind changing to a headwind can suddenly increase airspeed, potentially overwhelming the aircraft’s control systems.
Atmospheric Stability
The stability of the atmosphere can impact flight conditions. In a stable atmosphere, vertical air movement is restricted, which generally results in smooth flight conditions. In an unstable atmosphere, vertical movements increase, leading to turbulence, thunderstorms, and other adverse weather phenomena. Remote pilots should be mindful of the stability of the atmosphere during pre-flight planning.
Small Unmanned Aircraft Loading
Proper aircraft loading is a critical aspect of flight safety. Before any flight, the remote pilot-in-command (PIC) must ensure that the aircraft is correctly loaded and within its weight and balance limitations. Exceeding these limitations can lead to unsafe flight conditions, degraded performance, and increased risks during flight operations. The aircraft’s weight and balance data, provided by the manufacturer or builder, are essential references for determining whether an aircraft is properly loaded.
Weight and Its Impact on Aircraft Performance
Weight is a fundamental force that pulls the aircraft toward the Earth’s center due to gravity. The amount of lift generated by the aircraft must counteract its weight to achieve and maintain flight. Factors such as angle of attack (AOA), airspeed, and air density determine the amount of lift the aircraft can generate. If the aircraft is overloaded or improperly loaded, the lift may be insufficient to support the aircraft, resulting in a failure to take off or maintain stable flight.
Effects of Overloading
Overloading an aircraft has a direct and negative impact on almost every aspect of its performance. The key performance deficiencies of an overloaded aircraft include:
- Higher takeoff speed: The aircraft requires more speed to generate sufficient lift.
- Longer takeoff run: More runway or launch area is needed to achieve takeoff speed.
- Reduced climb rate and angle: The aircraft may struggle to gain altitude or climb at a steep angle.
- Lower maximum altitude: Overloading limits the aircraft’s ability to reach higher altitudes.
- Shorter range: Excess weight increases fuel or battery consumption, reducing the range of the aircraft.
- Reduced cruising speed: The aircraft flies more slowly due to the extra load.
- Reduced maneuverability: Heavier aircraft are less responsive to control inputs, making them harder to maneuver.
- Higher stalling speed: The aircraft is more prone to stalling because the increased weight requires more lift at lower speeds.
- Higher approach and landing speed: The aircraft requires more speed for landing, which increases the landing distance.
- Longer landing roll: Overloaded aircraft require more distance to come to a complete stop after landing .
Weight and Balance
Compliance with weight and balance limits is critical for flight safety. Operating above the maximum weight limitation can compromise the aircraft’s structural integrity and its ability to maintain safe flight. Similarly, operating outside the approved center of gravity (CG) limits results in control difficulties. The center of gravity is a point where the aircraft’s total weight is evenly balanced. It plays a crucial role in determining the aircraft’s stability and controllability.
When the CG is not properly balanced within the aircraft’s designated limits, the following can occur:
- Nose-heavy aircraft: When the CG is forward of the optimal point, the aircraft may tend to pitch downward, making it harder to climb or maneuver.
- Tail-heavy aircraft: When the CG is too far back, the aircraft may pitch upward, leading to unstable flight, reduced maneuverability, and a higher risk of stalling .
Load Factors and Structural Considerations
Load factor refers to the amount of force applied to the aircraft in relation to its weight. It is measured in Gs, where 1 G represents the force of gravity at rest. Load factors increase during maneuvers, especially turns, and steep banks, where additional stress is placed on the aircraft’s structure.
For example, in a steep 60° bank, the aircraft experiences a load factor of 2 Gs, meaning the structure is under twice the force of its weight. This can increase the stalling speed and reduce safety margins. Stalls occur when the angle of attack becomes too steep for the wing to generate lift, leading to an abrupt loss of altitude.
An understanding of load factors is essential for safe operation, as remote pilots must avoid overstressing the aircraft’s structure. Exceeding load limits, especially during tight turns or quick maneuvers, increases the risk of structural failure or an unexpected stall .
Emergency Procedures
Introduction
An emergency during a small unmanned aircraft system (sUAS) flight is an unexpected and often unforeseen event that can have serious safety implications. As a remote pilot in command (PIC), it is your responsibility to handle emergencies efficiently to minimize harm to people, property, and the aircraft itself. The Federal Aviation Administration (FAA) permits deviations from 14 CFR Part 107 regulations during an emergency to ensure a safe resolution to the situation. However, any deviation should be reported to the FAA if requested.
The remote pilot’s knowledge and preparedness are crucial when managing an emergency. This includes ensuring that all crew members are familiar with emergency procedures and that the aircraft is in optimal condition before flight.
Inflight Emergencies
Inflight emergencies can arise unexpectedly, and the remote pilot must be prepared to act decisively. The primary responsibility of the remote pilot is to ensure that the flight poses no hazard to other people or property. Common inflight emergencies include loss of communication, battery failure, motor failure, or weather-related issues that jeopardize the stability and control of the aircraft.
Key points for inflight emergencies include:
- Safety of the Flight: Before each flight, the remote pilot must assess the operational safety of the aircraft. This includes ensuring that the aircraft is airworthy and that there are no imminent risks to persons or property.
- Pre-Flight Inspection: Conducting a thorough pre-flight inspection is essential to minimize the risk of emergencies. Manufacturers often provide pre-flight checklists to assist with this, and for those that do not, it is the responsibility of the remote pilot to develop a checklist that ensures safe operation.
- Deviating from Regulations: During an emergency, the remote pilot may take whatever actions are necessary, even if those actions result in deviating from the rules of Part 107. For example, if a drone’s battery is on fire, the pilot may need to ascend the aircraft above the 400-foot altitude limit to find a safe landing zone. The pilot must focus on eliminating hazards to people or property, even if it involves temporary non-compliance with regulations.
- Post-Emergency Reporting: If the FAA (referred to as “the Administrator”) requests a report after an emergency, the remote pilot must provide an account of the events, including details on the emergency response actions.
Crew Member Briefings
When utilizing crew members, such as visual observers (VOs), or when allowing a non-certificated person to manipulate the flight controls, it is essential that they are briefed on emergency procedures prior to the flight. The briefing ensures that all crew members understand the following:
- The nature of the flight.
- Potential emergency situations that may arise during the flight.
- The specific actions they must take if an emergency occurs.
This collaborative preparation can greatly reduce confusion and improve response time in critical situations.
Crew Resource Management (CRM)
Crew Resource Management (CRM) is a set of training procedures that emphasize the effective use of all available resources, human, hardware, and information, to ensure safe and efficient flight operations. In the context of sUAS operations, CRM is critical as it fosters good communication, decision-making, and teamwork among the remote pilot and any supporting crew members, such as visual observers (VOs) or other ground personnel.
The FAA’s CRM guidelines are rooted in the principles developed for manned aviation, but they are equally applicable to sUAS operations, especially those involving complex missions or where crew coordination is necessary. CRM helps reduce errors, improve operational efficiency, and enhance safety, even for small crew configurations. This makes it particularly relevant for remote pilots, who are responsible for ensuring that every member of their team is briefed on their roles and emergency procedures before each flight.
Key Components of CRM
1. Communication
Effective communication is the backbone of CRM. It ensures that all crew members are on the same page, fully aware of their responsibilities, and able to convey important information quickly and clearly. For sUAS operations, communication can take several forms, including verbal briefings before the flight, visual signals during the operation, and continuous updates on flight status.
- Pre-flight briefings: The remote pilot should brief all crew members, especially visual observers, on the operational plan, potential risks, and emergency procedures. Everyone should be clear about their roles and responsibilities before the flight.
- In-flight communication: During the operation, ongoing communication helps ensure that the team can adapt to changing conditions or emergencies. This may involve calling out potential hazards or confirming operational changes.
- Post-flight debriefings: After the flight, crew members should discuss what went well and any issues that occurred, allowing the team to learn and improve for future operations.
2. Decision-Making
A core principle of CRM is the promotion of sound decision-making. Remote pilots often face situations that require quick thinking, risk assessment, and problem-solving. CRM encourages crew members to participate in decision-making processes, contributing their perspectives and information to support the remote pilot’s ultimate decisions.
- Risk assessment: Remote pilots should be proactive in identifying potential hazards before and during the flight. CRM fosters a team-oriented approach, where all crew members are encouraged to offer input and alert the remote pilot to any risks they observe.
- Problem-solving: When problems arise, such as unexpected weather changes or equipment malfunctions, CRM principles guide the crew to gather information, evaluate alternatives, and implement the best course of action. This collaborative approach can lead to better outcomes than a pilot acting alone.
3. Workload Management
Another vital aspect of CRM is workload management, which ensures that all necessary tasks are completed efficiently without overwhelming any one individual. For remote pilots, managing workload involves distributing tasks among crew members and pacing activities to avoid fatigue or task saturation.
- Task delegation: In an sUAS operation, the remote pilot might delegate responsibilities, such as monitoring airspace or checking equipment, to other crew members. By spreading out tasks, the pilot can focus on controlling the aircraft and managing the overall mission.
- Time management: CRM emphasizes the importance of anticipating high workload periods, such as takeoff and landing, and preparing in advance. This may involve checking weather updates, ensuring all equipment is functioning correctly, and confirming the status of the operational area.
4. Situational Awareness
Situational awareness is the ability to accurately perceive the current status of the flight, including environmental conditions, the state of the aircraft, and the location of potential hazards. CRM helps maintain situational awareness by encouraging crew members to remain alert and report any issues they observe.
- Monitoring: Crew members such as visual observers play a critical role in maintaining situational awareness by scanning the sky for other aircraft or obstacles and informing the remote pilot of any changes in the environment.
- Decision-making based on awareness: The remote pilot should continuously update their mental picture of the situation based on input from crew members and adjust the flight plan accordingly.
5. Human Factors
CRM also addresses human factors, which include any physiological or psychological issues that could impair the performance of the remote pilot or crew members. Fatigue, stress, and poor health can negatively impact decision-making and reaction time. CRM encourages the use of checklists like the IMSAFE checklist (Illness, Medication, Stress, Alcohol, Fatigue, Emotion) to ensure that all personnel are fit to perform their duties safely.
Part 107 Employment Trends
The drone pilot job market has grown rapidly in recent years as the technology has matured and found applications across various industries such as agriculture, construction, real estate, entertainment, law enforcement, and military use. The Federal Aviation Administration (FAA) began issuing Remote Pilot Certificates in 2016, and since then, the demand for licensed drone operators has surged.
Here’s a general overview of the employment trends in this sector:
- 2016: The year saw the introduction of FAA’s Part 107 regulation, which allowed commercial drone operations. The number of certified pilots quickly rose from a few thousand to around 20,000 by the end of the year.
- 2017-2018: During this period, the number of certified drone pilots in the U.S. grew significantly. By the end of 2018, the FAA reported more than 100,000 certified drone pilots. This rapid growth was driven by industries like real estate, agriculture, and inspections.
- 2019-2020: The growth trajectory continued, with more than 160,000 certified remote pilots by 2020. The commercial applications of drones expanded further into areas such as environmental monitoring, disaster response, and package delivery pilots in the testing phase by companies like Amazon and UPS.
- 2021-2023: The demand for drone pilots increased in various sectors. By the end of 2021, the number of licensed drone pilots had surpassed 250,000, according to FAA estimates. The COVID-19 pandemic also accelerated demand in sectors like deliveries and remote inspections, creating a new wave of opportunities.
- Future Outlook: The drone pilot job market is expected to continue growing, particularly with the development of urban air mobility and further integration of autonomous and AI-driven drone technologies. According to research forecasts, the global commercial drone market size is expected to grow from about $22 billion in 2020 to over $63 billion by 2028, suggesting a further rise in job opportunities.
However, exact year-by-year job numbers can vary significantly depending on the country, industry, and level of automation in the drone industry.
Industries That Hire Drone Pilots
Here’s an approximate breakdown of the major industries that hire drone pilots in the U.S. based on recent reports and job market trends:
Industry | Estimated Percentage of Drone Pilot Jobs |
---|---|
Agriculture | 25-30% |
Construction & Real Estate | 20-25% |
Media & Entertainment | 10-15% |
Energy & Utilities | 10-12% |
Public Safety & Emergency | 8-10% |
Logistics & Delivery | 5-8% |
Insurance | 5-7% |
Environmental Monitoring | 3-5% |
Mining & Surveying | 3-5% |
Security & Surveillance | 2-3% |
Part 107 Salary Trends
The highest-paying industries for drone pilots are generally those that require specialized skills, operate in high-risk environments, or demand advanced equipment and technology. These sectors often pay well due to the complexity of the work, safety concerns, and the value of the data gathered. Below are the top-paying industries for drone pilots:
Industry | Average Salary Range |
---|---|
Energy and Utilities | 80,000 USD - 120,000 USD |
Mining and Surveying | 70,000 USD - 100,000 USD |
Construction & Infrastructure | 60,000 USD - 90,000 USD |
Agriculture (Precision) | 50,000 USD - 80,000 USD |
Media & Entertainment | 50,000 USD - 100,000+ USD (per project) |
Logistics & Delivery | 50,000 USD - 80,000 USD |
Insurance | 60,000 USD - 85,000 USD |
Public Safety & Emergency | 50,000 USD - 90,000 USD |
Environmental Conservation | 50,000 USD - 75,000 USD |
As shown below, there has been a steady increase in the number of drones employed in the logistics sector, driven by the rise of e-commerce, last-mile delivery needs, and advancements in autonomous drone technologies. The trend is expected to continue as logistics companies increasingly adopt drones for faster and more efficient delivery solutions.
Radio Communication Procedures
Introduction
Radio communications play a pivotal role in maintaining the safety and efficiency of operations in the NAS. For manned aircraft, radio communication is used to share essential flight information with Air Traffic Control (ATC) and other aircraft. Although sUAS pilots are generally not required to use radios, understanding the structure and content of aviation communication is important for maintaining situational awareness. Remote pilots should be familiar with aviation language and radio protocols in case they need to operate near airports or monitor other aircraft operations for safety.
Understanding Proper Radio Procedures
Proper radio communication procedures allow for clear, concise, and unambiguous exchanges between pilots and ATC. The Federal Aviation Administration (FAA) uses standardized phraseology to avoid confusion and ensure that messages are interpreted correctly.
- Pilot/Controller Glossary: The Aeronautical Information Manual (AIM) contains a glossary of standard radio communication terms used between pilots and ATC. This glossary helps ensure consistency across all aviation communications, promoting a common understanding between all users of the airspace system.
- Phonetic Alphabet: The International Civil Aviation Organization (ICAO) phonetic alphabet is used in aviation communications to prevent misunderstanding of critical information like call signs, frequencies, and waypoint names. Remote pilots should be familiar with this alphabet, especially when monitoring communications or providing input in an emergency.
Phonetic Alphabet Example:- A = Alpha
- B = Bravo
- C = Charlie
- D = Delta
- E = Echo
- F = Foxtrot
Traffic Advisory Practices at Airports without Operating Control Towers
For airports without operating control towers, the responsibility for maintaining safety falls largely on the pilots. In these environments, pilots use Common Traffic Advisory Frequencies (CTAF) to announce their positions and intentions. CTAF allows pilots to self-announce their locations, providing critical situational awareness for other aircraft operating in the same vicinity.
- Communication Practices at Non-Towered Airports:
- MULTICOM: If no specific frequency is listed, the frequency 122.9 MHz is used for general traffic advisory.
- UNICOM: At airports with a UNICOM station, a pilot can request services like fuel or parking information. However, radio communications here are not managed by ATC.
- Recommended CTAF Broadcast Procedures: When approaching a non-towered airport, pilots typically make an initial broadcast when 10 miles from the airport. This broadcast includes their position and intention to enter the traffic pattern.
Example Broadcast:- “Town and Country traffic, Cessna 123 Bravo Foxtrot is 10 miles south, inbound for landing, Town and Country traffic.”
Communication at Towered Airports
At airports with operating control towers, radio communications are managed by ATC, which is responsible for providing clearances, sequencing arrivals and departures, and ensuring separation between aircraft. Remote pilots operating under Part 107 must receive explicit authorization to operate in controlled airspace, usually through the Low Altitude Authorization and Notification Capability (LAANC) system. While sUAS pilots may not directly communicate with ATC, monitoring relevant frequencies can improve situational awareness.
- Call Signs: All aircraft have unique call signs, typically beginning with an “N” in the United States (e.g., N123AB). Larger airlines use their company name followed by a flight number (e.g., “Delta 715”).
- Radio Transmissions: ATC communications include vital information about runway usage, aircraft separation, and other safety measures. Understanding how ATC manages airspace, even passively, helps remote pilots avoid conflicts.
Emergency Situations
Remote pilots are generally not expected to transmit over aviation frequencies unless an emergency arises. However, if such a situation occurs, understanding radio phraseology and etiquette will allow the pilot to effectively communicate with other aircraft or ATC to resolve the emergency safely. In such cases, the pilot should clearly announce their call sign, position, and nature of the emergency.
Determining the Performance of Small Unmanned Aircraft
Aircraft performance refers to how well an aircraft can achieve various operational tasks, including takeoff, climb, cruising, and landing. For small unmanned aircraft systems (sUAS), performance is influenced by a number of environmental and mechanical factors. The manufacturer typically provides performance data to help operators predict how their aircraft will behave under specific conditions. This data includes information on takeoff distance, climb rate, payload capacity, maximum altitude, and more.
While manufacturer data is often the best resource for assessing performance, remote pilots should also consider factors such as temperature, altitude, and humidity, as these variables can alter performance significantly. In the absence of specific data from the manufacturer, remote pilots should seek information from trusted sources or other users of the same aircraft model.
Effects of Temperature on Performance
Temperature has a direct effect on air density, which, in turn, affects how efficiently an aircraft can generate lift. As temperature increases, the density of the air decreases, which means that the aircraft has to work harder to produce the lift required for flight.
- High Temperatures: In warmer conditions, the air is less dense, resulting in a decrease in lift. As a result, the aircraft may require a longer distance for takeoff and a reduced rate of climb. Battery efficiency may also be affected, reducing the drone’s range and endurance.
- Low Temperatures: In cooler conditions, the air is denser, allowing the aircraft to produce more lift and operate more efficiently. Cold air improves overall performance, including shorter takeoff distances, faster climb rates, and increased payload capacity.
Effects of Humidity on Performance
Humidity, or the amount of water vapor in the air, also affects air density. Water vapor is lighter than dry air, meaning that humid air is less dense than dry air. While humidity alone is not usually a primary factor in performance calculations, it can contribute to a higher density altitude, thereby affecting the aircraft’s ability to generate lift.
- High Humidity: In humid conditions, the aircraft may experience reduced performance, similar to the effects of high temperatures. Higher humidity leads to thinner air, requiring more power to maintain lift and causing longer takeoff distances.
- Low Humidity: Dry air, being denser, enhances performance. The aircraft will be able to generate more lift and climb more efficiently in low-humidity conditions.
Key Performance Factors
Several factors related to weight, altitude, and configuration can influence the performance of a small unmanned aircraft:
- Takeoff and Landing Distance: In conditions with high temperature, humidity, or altitude, the takeoff and landing distance required by the aircraft will increase. As air density decreases, the aircraft requires more speed to generate the same amount of lift, resulting in longer distances for takeoff and landing.
- Rate of Climb: The rate of climb is directly affected by the aircraft’s ability to generate excess thrust or power. As weight, altitude, or environmental conditions like high temperature increase, the rate of climb decreases. Climb performance diminishes as the air becomes less dense at higher altitudes, or as the aircraft becomes heavier.
- Payload and Range: The more weight (payload) added to the aircraft, the more energy it requires to stay airborne, which reduces its range and fuel or battery efficiency. Manufacturers often specify maximum payload capacities, and exceeding these limits can cause the aircraft to lose stability or fail to climb adequately.
Density Altitude
Density altitude is a critical concept for understanding how environmental conditions affect aircraft performance. Density altitude is a theoretical altitude calculated based on temperature, pressure, and humidity, which correlates to how the aircraft performs. As air density decreases, the density altitude increases, meaning the aircraft behaves as though it is flying at a higher altitude, even if it is physically closer to the ground.
- High Density Altitude: When the density altitude is high (caused by high temperatures, high humidity, or low pressure), aircraft performance is reduced. The drone will require more power for the same maneuvers, climb rates will diminish, and battery or fuel consumption will increase.
- Low Density Altitude: In cooler, dry conditions with higher air pressure, density altitude is low, and the aircraft will perform more efficiently. Climb rates will improve, and takeoff distances will be shorter.
Physiological Factors (Including Drugs and Alcohol) Affecting Pilot Performance
Introduction
Operating a small unmanned aircraft requires peak physical and mental performance. Many physiological factors, including illness, medication, stress, alcohol, fatigue, and emotion, can affect a pilot’s ability to perform optimally and make sound decisions. Remote pilots must be aware of these factors to prevent impaired performance that could jeopardize flight safety. Before any flight, the pilot should conduct a self-assessment using the IMSAFE checklist, which stands for:
- Illness: Am I sick?
- Medication: Am I taking any medications that could impair my abilities?
- Stress: Am I under stress that could impact my focus?
- Alcohol: Have I consumed alcohol within the last 8 hours?
- Fatigue: Am I tired and not adequately rested?
- Emotion: Am I emotionally upset or distracted?
This self-assessment ensures that the pilot is fit to fly and not at risk of impairment due to any of these factors.
Hyperventilation
Hyperventilation occurs when a person breathes too quickly, expelling excessive amounts of carbon dioxide. This condition is often triggered by stress or anxiety, and while it may not fully incapacitate a pilot, it can result in disturbing symptoms such as:
- Visual impairment
- Unconsciousness
- Dizziness
- Tingling sensations
- Muscle spasms
To prevent or manage hyperventilation, it’s essential to breathe slowly and deeply or breathe into a paper bag to restore the carbon dioxide levels in the bloodstream. Hyperventilation is more likely to occur in stressful situations, so pilots must remain calm and in control.
Stress
Stress is the body’s reaction to physical, psychological, or emotional demands. It can trigger the release of hormones like adrenaline, increasing heart rate, blood pressure, and alertness. There are two main types of stress that can affect pilot performance:
- Acute Stress: A short-term response to immediate danger or pressure, which can be manageable in small doses. However, if it accumulates, it can lead to degraded performance.
- Chronic Stress: This is a prolonged condition resulting from unresolved stressors. Pilots suffering from chronic stress may experience persistent fatigue, irritability, and reduced cognitive function, making them unsafe to fly.
Both forms of stress can significantly impair decision-making and concentration, which are vital to safe flight operations. Pilots experiencing high stress should seek medical advice and avoid flying until the situation is under control.
Fatigue
Fatigue is a common factor contributing to pilot error and affects attention, concentration, and coordination. Fatigue can be physical, due to lack of rest, or mental, resulting from long periods of cognitive effort or stress. It’s critical to distinguish between two types of fatigue:
- Acute Fatigue: Short-term fatigue caused by a period of strenuous effort, excitement, or lack of sleep. Rest and proper sleep usually alleviate acute fatigue.
- Chronic Fatigue: Long-term fatigue, which persists over time due to psychological or physical factors, such as constant stress or a medical condition. Chronic fatigue can lead to physical and emotional health problems and requires medical intervention.
Symptoms of fatigue include a decrease in performance, slower reaction times, and impaired judgment. Pilots should avoid flying if they have not had adequate rest, are feeling fatigued, or have been working excessively long hours.
Dehydration
Dehydration results from a critical loss of water in the body and can significantly impact pilot performance. It often occurs in hot conditions or when pilots consume diuretic beverages like caffeinated drinks and alcohol. Symptoms include:
- Headache
- Fatigue
- Cramps
- Dizziness
- Nausea
To prevent dehydration, pilots should drink two to four quarts of water every day, especially when flying in hot conditions. It’s important to remain hydrated even before thirst sets in, as dehydration can cause fatigue and impair decision-making abilities.
Heatstroke
Heatstroke is a severe condition resulting from the body’s inability to regulate its temperature. It can occur due to prolonged exposure to high temperatures, especially in combination with dehydration. Symptoms include:
- Fatigue
- Nausea
- Dizziness
- Complete collapse
To avoid heatstroke, pilots should ensure proper hydration and take breaks in shaded or cooler environments if operating in extreme heat. If heatstroke symptoms appear, immediate medical attention is necessary.
Effects of Alcohol on Pilot Performance
Alcohol has a profound impact on the body, and even small amounts can impair a pilot’s ability to operate an sUAS safely. According to 14 CFR Part 107, a pilot cannot operate a small UA if they:
- Have consumed any alcohol within the preceding 8 hours.
- Are under the influence of alcohol.
- Have a blood alcohol concentration (BAC) of .04 percent or greater.
- Are using any drugs that impair mental or physical capabilities.
Alcohol impairs judgment, coordination, reaction time, and memory, all of which are critical for safe flight operations. Even one drink can affect the speed and accuracy of decision-making. It is also important to recognize that a hangover can leave residual alcohol in the body, impairing a pilot even if they believe they have fully recovered. It can take over 16 hours for significant amounts of alcohol to leave the body.
Drugs
Pilots should avoid operating under the influence of any drugs, including over-the-counter (OTC) medications, which may cause drowsiness or impair cognitive function. Antihistamines, decongestants, and certain pain medications are common culprits that can reduce alertness. Before using any medication, pilots should wait at least 48 hours to ensure no adverse effects occur. It is critical to read the label of any medication and consult a physician if unsure about its potential impact on flight performance.
Aeronautical Decision-Making and Judgment (ADM)
Aeronautical Decision-Making (ADM) is a systematic approach to assessing and managing risks during flight operations. ADM involves recognizing personal attitudes and external factors that can affect a pilot’s judgment and implementing strategies to mitigate those risks. The FAA recognizes that approximately 80 percent of aviation accidents are due to human error, often stemming from poor decision-making, making ADM a vital aspect of flight safety.
Incorporating ADM into sUAS operations can reduce the likelihood of errors by enhancing the pilot’s ability to evaluate risks, manage stress, and make well-informed decisions. ADM provides a framework for consistently analyzing situations, improving safety, and making sound choices.
History of ADM
ADM has been a focal point in aviation training for over 25 years, initially developed to reduce accidents caused by human factors in manned aviation. The FAA’s formal ADM training materials were created to teach decision-making as part of pilot training and certification. Research has shown that pilots trained in ADM make fewer inflight errors, leading to a marked reduction in accidents.
ADM is now a required subject in remote pilot certification under FAA Part 107. Understanding and applying ADM concepts are essential for sUAS pilots operating in the National Airspace System (NAS), especially when making critical decisions during flight operations.
Steps for Good Decision-Making in ADM
- Identify Personal Attitudes: Personal attitudes can lead to poor decision-making. Identifying hazardous attitudes such as invulnerability, impulsivity, or resignation is the first step in recognizing behaviors that may compromise flight safety.
- Learn Behavior Modification Techniques: Once hazardous attitudes are identified, the next step is to apply antidotes. For example, an antidote for impulsivity (the urge to act without thinking) might be “Think first.”
- Stress Management: Stress can negatively impact decision-making. Learning techniques to manage stress, such as regular relaxation or fitness activities, can improve mental clarity during flight.
- Risk Assessment Skills: Pilots must evaluate the risks associated with each flight. Understanding the trade-offs between risk and reward is essential for minimizing potential hazards.
- Using All Available Resources: sUAS pilots should utilize all available resources, including crew members, technology, and environmental information, to enhance situational awareness.
- Evaluate ADM Skills: Post-flight evaluations are key to assessing the effectiveness of the ADM process and improving future decision-making capabilities.
Risk Management
ADM is closely tied to risk management, a process that identifies hazards and mitigates risks to ensure safe flight operations. Risk management follows four key principles:
- Accept No Unnecessary Risk: Every flight involves inherent risks, but avoidable risks should be eliminated. For example, flying in poor weather conditions without necessity adds undue risk.
- Make Risk Decisions at the Appropriate Level: Decisions should be made by the pilot with the ability to implement risk controls.
- Accept Risk When Benefits Outweigh Costs: Sometimes risk is necessary, but only when the potential benefits justify the danger.
- Integrate Risk Management at All Levels: Risk management should be part of every phase of flight planning and operation, from pre-flight assessments to inflight adjustments.
Decision-Making Models
ADM relies on structured decision-making models that help pilots evaluate risks, process information, and make effective decisions. Two common models used in ADM are:
3P Model:
- Perceive: Identify the present circumstances and any potential hazards (e.g., weather conditions, obstacles).
- Process: Evaluate the significance of these factors in terms of flight safety.
- Perform: Implement the best course of action to mitigate risk.
DECIDE Model:
- Detect: Recognize that a change has occurred.
- Estimate: Evaluate the need to counter or react to the change.
- Choose: Select a desirable outcome.
- Identify: Identify necessary actions to correct the issue.
- Do: Take action.
- Evaluate: Assess the result of the action and adjust as needed.
These models provide a systematic approach to decision-making and ensure pilots take deliberate steps to mitigate risks in real-time.
Operational Pitfalls
Even experienced pilots can fall into operational pitfalls, which are behavior patterns or tendencies that compromise safety. Common pitfalls include:
- Get-there-itis: The desire to complete a mission at the expense of safety.
- Macho Attitude: The desire to impress others or prove skill, leading to unsafe actions.
- Resignation: A passive attitude where the pilot believes they have no control over the outcome, leading to inaction.
By recognizing these pitfalls and applying ADM principles, pilots can avoid dangerous situations and make safer decisions.
Airport Operations
Airport operations are a critical area of knowledge for remote pilots to ensure safe and effective flight, especially when flying in or near airports. An airport is any land or water area used for landing and takeoff of aircraft, and it includes surrounding facilities such as buildings and navigational aids. Remote pilots must be familiar with airport operations to ensure that their small unmanned aircraft system (sUAS) flights do not interfere with manned aircraft and other airport activities.
There are two main types of airports: towered and non-towered. Understanding how these airports function and the rules for flying near them is essential to avoid collisions and disruptions in airspace.
Types of Airports
- Towered Airports:
Towered airports have an operating control tower that provides air traffic control (ATC) services to ensure safe, orderly, and efficient management of air traffic. ATC manages aircraft movements on the ground and in the surrounding airspace. Remote pilots are required to obtain prior authorization from ATC before flying in controlled airspace around towered airports, typically through systems like LAANC (Low Altitude Authorization and Notification Capability). - Non-Towered Airports:
Non-towered airports do not have an operating control tower, which means pilots manage their own aircraft movements using visual flight rules (VFR) and by communicating with each other via a Common Traffic Advisory Frequency (CTAF). Remote pilots flying near non-towered airports should monitor CTAF to understand the positions and intentions of manned aircraft.
Remote pilots should be aware that although two-way radio communication is not required at non-towered airports, it is highly recommended for improved situational awareness. At these airports, remote pilots must yield right of way to manned aircraft and follow established traffic patterns. - Subcategories of Airports:
- Civil Airports: Open to the general public.
- Military/Federal Government Airports: Operated by the military, NASA, or other federal agencies.
- Private Airports: Restricted-use airports that are not open to the public and require permission for access.
Traffic Patterns at Non-Towered Airports
At non-towered airports, it is critical to understand the traffic patterns used by manned aircraft. These traffic patterns guide the safe flow of traffic during landings and takeoffs. Aircraft typically follow a rectangular pattern at a prescribed altitude to organize their approach and departure from the airport.
- Entry into the Pattern: Aircraft should enter the traffic pattern at a 45-degree angle to the downwind leg of the pattern, ideally at the midpoint.
- Pattern Altitude: Remote pilots must familiarize themselves with the pattern altitude, as this is where most manned aircraft operate when approaching or departing the airport. Maintaining situational awareness at this altitude is critical to avoid conflicts with manned aircraft.
Sources for Airport Data
Before flying near any airport, remote pilots should gather up-to-date information on the airport’s operations, services, and potential hazards. The following are key sources of airport data:
- Aeronautical Charts: Aeronautical charts provide essential information about the airport and surrounding airspace. These charts are essential for identifying airspace classifications, restricted areas, and airport locations. Sectional charts and VFR Terminal Area charts are the most commonly used charts for these purposes.
- Chart Supplement U.S. (formerly known as the Airport/Facility Directory): The Chart Supplement U.S. provides detailed information about airports, heliports, and seaplane bases that are open to the public. This resource is updated every 56 days and includes details on communication frequencies, runway lengths, airport services, and other critical operational data. Remote pilots should consult this supplement for comprehensive information about any airport they intend to fly near.
- NOTAMs (Notices to Airmen): NOTAMs are time-critical updates that provide information on temporary changes affecting airports, such as runway closures, construction, or other hazards. Before any flight, pilots should check for relevant NOTAMs that could affect their operation. Remote pilots can use NOTAMs to ensure they are aware of any changes to airport procedures or infrastructure.
- Automated Terminal Information Service (ATIS): ATIS is a recorded broadcast that provides updated weather conditions, active runways, and other important non-control information at towered airports. Remote pilots can monitor ATIS to stay informed of current conditions and adjust their operations accordingly. The broadcast is updated regularly, often once per hour, but can be updated more frequently if conditions change.
Latitude and Longitude
Understanding latitude and longitude is essential for interpreting aeronautical charts and determining precise geographical locations for flight operations. Latitude lines run east-west and are measured in degrees north or south of the equator. Longitude lines run north-south, measured in degrees east or west of the Prime Meridian. Together, these coordinates allow remote pilots to pinpoint specific locations with accuracy, ensuring that flights remain within authorized areas and avoid restricted zones.
Antenna Towers and Other Obstacles
Flying near airports often involves dealing with various obstacles, such as antenna towers. Remote pilots should exercise caution when flying near structures, particularly those that exceed 1,000 feet above ground level (AGL). These structures, often supported by guy wires, can be difficult to see and may pose significant hazards. It is recommended to avoid these obstacles by at least 2,000 feet horizontally to ensure safety.
Maintenance and Preflight Inspection Procedures
Proper maintenance and preflight inspections are critical to ensuring the safe operation of a small unmanned aircraft system (sUAS). According to the Federal Aviation Administration (FAA), remote pilots are responsible for ensuring that their aircraft is in a safe operating condition before each flight. This responsibility includes regularly scheduled maintenance as well as thorough preflight inspections. Failing to adhere to proper maintenance and inspection procedures could lead to mechanical failures, loss of control, or accidents that may result in damage to property or injury to people.
The FAA provides guidelines for maintenance and inspection under 14 CFR Part 107, emphasizing that each aircraft must be maintained in accordance with manufacturer guidelines or, in the absence of such guidelines, in accordance with the best practices outlined by the FAA. Both maintenance and preflight inspections should be considered essential parts of flight safety operations.
Maintenance Procedures for sUAS
The maintenance of small unmanned aircraft systems (sUAS) is critical for ensuring their reliability and longevity. The FAA recommends following a proactive approach to maintenance, whether through following manufacturer’s guidelines or by adopting best practices developed by the remote pilot or operator. Regular maintenance tasks may include:
- Battery Maintenance: sUAS systems rely heavily on battery power, and maintaining battery health is critical to ensuring a safe and successful flight. Batteries should be checked for wear and damage, and charging cycles should be carefully monitored to ensure proper function. Overcharging, undercharging, or storing batteries improperly can reduce their life span or cause sudden failures during flight.
- Propeller Inspection and Replacement: Propellers are key components that provide thrust, and they are subject to wear and damage. Regular inspection for chips, cracks, and other signs of wear is crucial. If any damage is found, the propellers should be replaced immediately to prevent loss of control during flight.
- Firmware and Software Updates: Remote pilots must ensure that the aircraft’s firmware and control software are up to date. These updates often contain important fixes for bugs or new features that can enhance safety and performance. Keeping all system components updated is vital to avoiding technical issues.
- Airframe Integrity: The structural integrity of the sUAS airframe must be checked for signs of damage, including cracks, warping, or loose components. These inspections are particularly important after a hard landing or collision, as damage may not always be visible but can still compromise the aircraft’s safety.
Preflight Inspection Procedures
Preflight inspections are a critical step in ensuring the safety and reliability of an sUAS before each operation. Remote pilots should develop and follow a checklist that includes all the necessary components for a thorough inspection. The FAA emphasizes that preflight inspections should always be conducted before the aircraft is flown, regardless of whether the system was previously inspected.
Key components of a preflight inspection include:
- Visual Inspection: A thorough visual inspection of the aircraft is necessary to identify any obvious signs of damage or wear. This includes checking the propellers, motors, airframe, and landing gear for cracks, loose connections, or damage that could affect performance.
- Battery Check: Batteries should be fully charged and securely mounted. The pilot should also check for any signs of swelling, leaks, or other damage that could lead to a failure during flight. Batteries that show signs of wear or damage should be replaced immediately.
- Control System Check: The remote control or ground station that will be used to operate the aircraft must also be inspected. This includes checking that the control sticks and switches function properly, that the antennas are intact, and that the system can communicate with the aircraft without issues.
- Firmware and Software Validation: The aircraft’s firmware should be up to date, and the pilot must confirm that the software has not been corrupted. Ensuring the aircraft’s software and ground control system are synced is essential for reliable operation.
- GPS and Navigation System Verification: Pilots should ensure that the GPS and any other navigation systems are functioning properly. Any discrepancies in the system could result in loss of control or miscommunication between the pilot and the aircraft.
- Weather Conditions Assessment: A preflight inspection is not complete without assessing the weather conditions, which can greatly affect flight safety. Pilots should check for any environmental factors such as high winds, precipitation, or low visibility that could hinder safe flight operations.
Importance of Preflight Checklists
A preflight checklist ensures that all critical aspects of the aircraft’s condition and readiness for flight are examined systematically. By following a checklist, remote pilots minimize the risk of overlooking a crucial component. Checklists are especially important when working in high-stress environments, as they help to ensure consistency and thoroughness.
For aircraft that do not come with manufacturer-provided checklists, the FAA advises pilots to develop their own checklist. This checklist should cover all key systems, including mechanical components, software, and environmental conditions. Each item should be carefully reviewed and confirmed before flight to reduce the risk of failure or accident during the operation.